top of page

-WELCOME-
Our research focuses on quantum optics and quantum information science.
If you are interested in our group, contact us
-Latest Publications-
-
Observation of Highly Correlated Ultrabright Biphotons Through Increased Atomic Ensemble Density in Spontaneous Four-Wave Mixing
-
Quantum interface for telecom frequency conversion based on diamond-type atomic ensembles
-
Controlling frequency-domain Hong-Ou-Mandel interference via electromagnetically induced transparency
-
Quantum fidelity of electromagnetically induced transparency: the full quantum theory
About Our Research
Cold atoms produced by magneto-optical trap [1] is a very clean quantum matter that can be used to study quantum optical phenomena such as electromagnetic induced transparency (EIT) [2]. The following are the main projects of our research, each of which contains theoretical and experimental parts.
Biphoton Generation

Photons are ideal carriers for quantum information science because of their fast propagation speed and weak interaction with the environment. Here, we use the spontaneous four-wave mixing in cold Rb atoms to generate the correlated photon pairs [3]. The photon pairs are polarization entangled due to the indistinguishable nature of the dipole transition paths in the sub-Zeeman levels. Unlike the common method of using spontaneous down-conversion to produce THz-bandwidth single photons in solid crystals, the new method we use produces photon pairs with only a few MHz bandwidth or less, which is advantageous in quantum information applications.
Quantum Frequency Converter
A key topic in optical quantum computing and communication is to convert the frequencies of individual photons with little to no loss for transporting information among various types of quantum devices [4,5].
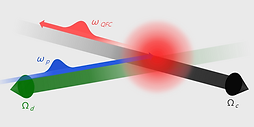
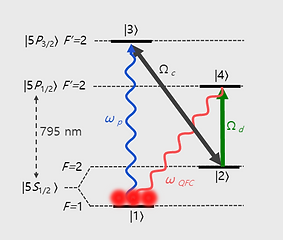
To this end, we use EIT-based backward-configuration four-wave mixing in cold Rb atoms [6]. According to the theoretical model, the conversion efficiency (CE) can be close to 100% under ideal conditions. In the experiment, we currently achieve around 70% frequency conversion between 780nm and 795nm. Next we intend to increase the optical density (OD) to obtain at least 90% CE of the single photon state.
Quantum Phase Gate
In quantum information science, the realization of large cross-phase modulations (XPM) at the single-photon level is an important but challenging task. Here, we propose a novel XPM scheme based on a phase-dependent double-Λ EIT system. With this scheme, we observe a large cross-phase shift of 3.6 radians induced by a light pulse containing around eight photons in cold Rb atoms [7]. This XPM scheme does not require cavities or Rydberg atoms, which provides a simple route to generate strong interactions between photons and obtain large cross-phase shifts per photon. According to theoretical model, the proposed XPM scheme can reach the goal of single-photon π phase modulation when using two phase-coherent single-photon pulses.
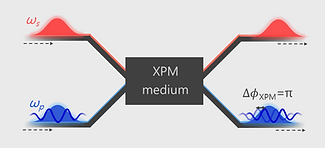
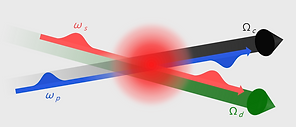
Quantum State Tomography

Imagine that we received a photon source. Can we "recognize" the source as coherent, Fock, thermal, or even a mixture of the above? A method called quantum state tomography (QST) [8] using balanced homodyne detection can reconstruct the detailed features of the photon state. This reconstructed photon state "fingerprint" has a special name called the Wigner function. Compared to the cold atomic system above, the QST setting is relatively small, but more sensitive to the environment. Therefore, in order to make the reconstruction as identical as possible to the original state, people must not only try to reduce the electronic, environmental, and other unwanted noises, but also use some special statistical methods to carefully analyze the data.
Other Researches
In addition to these major projects, our group has some minor research, including theoretical and experimental quantum mechanics issues listed below.
-
Weak measurement
-
Quantum Zeno effect
-
DLCZ protocol
-
Quantum memory
-
HOM interference
-
Quantum repeater
BIBLIOGRAPHY
[1] S. Chu, Rev. Mod. Phys. 70, 685 (1998).
[2] S. E. Harris, Phys. Today 50, 36 (1997).
[3] P. Kolchin, Phys, Rev. A 75, 033814 (2007).
[4] R. H. Hadfield, Nat. Photon. 3, 696 (2009).
[5] N. K. Langford et al., Nature 478 360 (2011).
[6] Z.-Y. Liu et al., Sci. Rep. 7, 15796 (2017).
[7] Z.-Y. Liu et al., Phys. Rev. Lett. 117, 203601 (2016).
[8] A. I. Lvovsky and M. G. Raymer, Rev. Mod. Phys. 81, 299 (2009).
bottom of page